2016年12月8日AMS发布会在欧洲核子中心举行,阿尔法磁谱仪(AMS)项目负责人、诺贝尔奖获得者丁肇中教授介绍了AMS实验在国际空间站上运行五年的主要科学成果。
阿尔法磁谱仪(AMS,图1)是在太空中运行的最灵敏的粒子探测器,它在物理学研究的未知领域和科学前沿进行着探索。AMS的独特之处在于它身处太空之中直接测量宇宙中的带电粒子和核子。相对于以往的实验而言,AMS极大地提高了测量的准确度和精确度。AMS运行时间长、接受度大、系统冗余可靠,以及在CERN进行的细致的束流测试使得AMS的测量精度达到1%的水平。已经在国际空间站上运行5年的AMS所获取的数据正在为我们解开宇宙的奥秘。
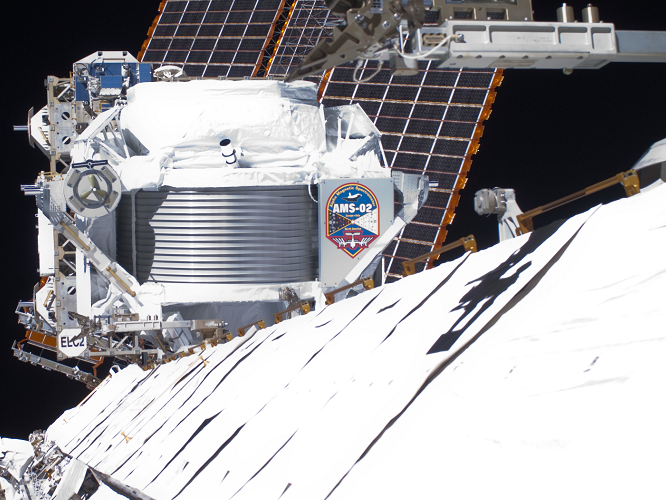
图1. 在地球上方240英里(400公里)的太空中,阿尔法质谱仪(AMS)探测着未与地球大气层发生相互作用的宇宙中的原初宇宙线。
自从2011年5月安装在国际空间站上以来,AMS已经收集了超过900亿宇宙线,并在物理评论快报上发表了主要物理结果。
初级宇宙线由超新星爆炸产生,它们在到达AMS之前已经在太空中旅行了几百万年。次级宇宙线是由初级宇宙线与恒星际物质相互作用而产生的。处于国际空间站上独一无二的位置,AMS通过精密的探测器测量宇宙线的电荷、能量和动量,从而让我们能有机会更深入地了解暗物质,寻找宇宙中的原初反物质,测量初级与次级宇宙线的特征以及搜寻潜在的新物理现象。
宇宙中有数百种带电基本粒子。其中的电子、质子、正电子以及反质子具有无限长的寿命,它们可以在宇宙中一直传播并最终穿过AMS探测器。AMS的测量结果显示,电子流强与正电子流强的强度不同,随能量变化的行为也不一样。令人惊奇的是,在60GeV到500GeV的能量范围内,正电子、质子和反质子的流强显示出一样的随动量变化的行为,但电子的表现则完全不同,如图2所示。这是一个出人意料的发现,因为电子与正电子在恒星际磁场中损失等量的能量,而且比质子和反质子的能量损失要大得多。
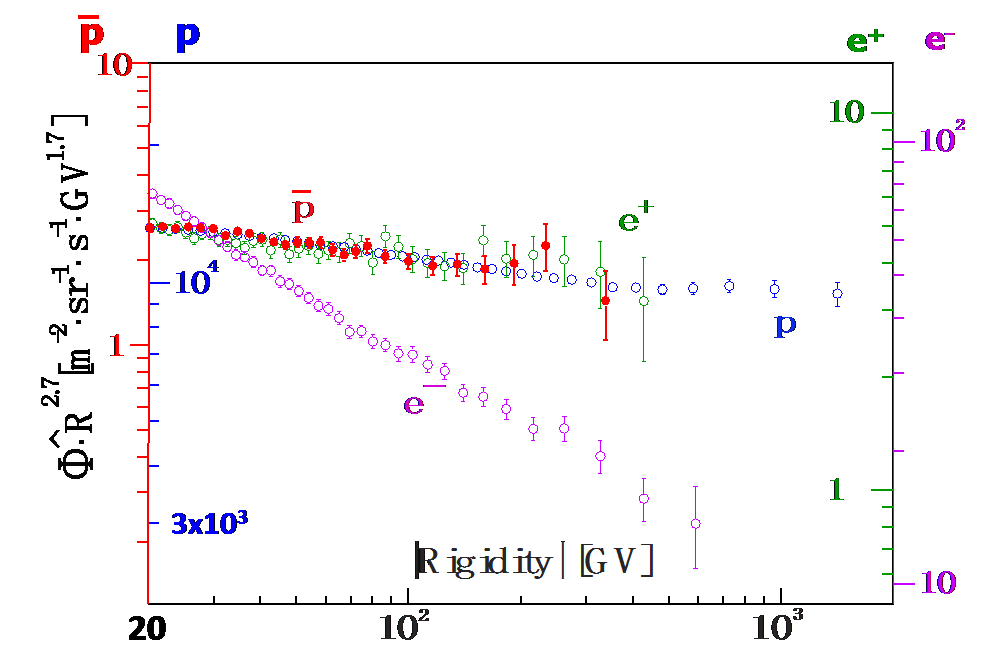
图2. 在60GeV到500GeV能量范围内,正电子、质子和反质子的能谱显示出一样的随动量变化的行为,而电子的行为则完全不同,随着动量的增加,电子流强更快速地下降。
过去的几十年中,人们非常关注对暗物质的性质以及起源的研究。暗物质粒子相互碰撞会产生能量,进而转化成普通的粒子如正电子、反质子。暗物质的特征信号是粒子流强随能量增加而出现超出,然后在与暗物质质量相应的能量以上急剧的下降。
如图3所示,从8GeV开始在传统宇宙线碰撞模型的基础上有所超出,之后在高能量处显示出急剧减少的趋势。正电子的数据能够很好地与暗物质质量为1TeV的暗物质模型相吻合。AMS对宇宙线基本粒子的探测引起了物理学界广泛的兴趣。其他可以解释AMS正电子流强超出与急剧下降的模型认为正电子来源于如脉冲星以及超新星爆炸的天体物理现象,但这些模型并不能对AMS其他粒子的精确测量结果进行解释。
同时,AMS测量了反质子-质子流强比例 ,该结果测量到的超出的反质子不可能来自于脉冲星。
图3. AMS正电子流强最新结果与理论模型相比较
质子是宇宙线种最丰富的粒子。AMS能够以1%的精度精确测量质子流强。所获得的数据结果显示,现有的理论无法描述质子流强。
同时,AMS研究了氦、碳、氧等轻原子核和以及重至铁核的较重原子核。人们通常认为,氦、碳和氧是初级宇宙线,直接在超新星爆炸中产生;而锂、铍和硼是次级宇宙线,由初级宇宙线与恒星际物质碰撞产生。初级宇宙线携带了关于宇宙线起源和传播的信息,次级宇宙线携带了关于初级和次级宇宙线传播以及星际物质的信息。
虽然锂是次级宇宙线,它的能谱的行为与质子、氦子相似,这三种核子的能谱都不能被现有的模型所解释。
因为质子、氦、碳和氧是初级宇宙线,产生于相同的宇宙线源,所以他们的流强应该是与刚度无关的。刚度等于单位电荷下的动量,它决定了宇宙线所经过的磁场对宇宙线粒子的偏转效应。,AMS测量的碳-氦流强比和氧-碳流强比是与刚度无关的。但出乎意料的是, 质子-氦流强比却随着刚度的增加而迅速但平滑的下降。
AMS也测量了其他的次级宇宙线,包括硼(B)和铍(Be)。铍的不稳定同位素,铍-10,会衰变到硼,半衰期为150万年。由于接近光速的铍-10核子的相对论时间膨胀效应,随着能量的增加,铍-硼流强比例增加 。因此,对铍-硼流强比例进行探测能够得到关于宇宙线在星系间传播时间的信息。通过这一测量方法,AMS测得银河系宇宙线的年龄大约是1200万年。
次级宇宙线(如硼)与初级宇宙线(如碳)的流强比,提供关于宇宙线传播和传播过程中所穿越的恒星际物质的信息。通常人们以快速移动气体在磁化等离子体中的扩散过程来建立宇宙线传播的模型。不同的磁化等离子体模型预言的硼-碳流强比具有不同的特征。出人意料的是,在65 GV以上,AMS测量的硼-碳流强比(B/C)可以用单一幂律谱(B/C=kRd )来描述,其中d = -0.333±0.015。这一结果符合柯尔莫哥洛夫的磁化等离子体湍流模型的预言,即d渐近为-1/3。同样重要的是,硼-碳流强比没有任何显著的结构,这与很多声称可以解释AMS所测量到的正电子能谱的行为的宇宙线模型所预言的不同。
AMS的测量表明,初级宇宙线中的碳和氧,与次级宇宙线中的硼、锂和铍相比, 他们的流强随动量(或刚度)的变化显示出明显不同的特征。
宇宙大爆炸起源模型认为在宇宙极早期物质和反物质的数量是相等的,但实际却无法观测到原初反物质,而这一现象被称为重子数产生过程。重子数产生过程要求强的对称性破坏和有限的质子寿命。在过去的半个世纪里,尽管科学家们在实验室中花费了大量的精力,仍然无法获得存在强的对称性破坏和质子衰变的证据。因此,在宇宙线中观测到单个反氦核事件有着非常重大的意义。
AMS已经收集到了37亿个电荷为+2的氦核事例,此外还观测到几个电荷为-2、质量在3He范围内的特殊事例。当候选事例率为每年约1个并且信号(反氦候选事例)/本底(氦)的排除率要求达到1/10亿的时候,我们对探测器的理解就更为重要。在未来的日子里,我们将收集更多的数据来确定这些反氦候选事例的来源。
在国际空间站上运行五年以来,AMS探测到了宇宙线基本粒子与核子的精确信息,正在逐渐为我们解开宇宙的奥秘。
附录1
AMS 在物理评论快报上发表的主要结果
“First Result from the Alpha Magnetic Spectrometer on the International Space Station : Precision Measurement of the Positron Fraction in Primary Cosmic Rays of 0.5-350 GeV”, M. Aguilar et al., Phys. Rev. Lett. 110, 141102 (2013) (Selected as Editors’ Suggestion).
“High Statistics Measurement of the Positron Fraction in Primary Cosmic Rays of 0.5-500 GeV with the Alpha Magnetic Spectrometer on the International Space Station”, L. Accardo et al., Phys. Rev. Lett. 113, 121101 (2014) (Selected as Editors’ Suggestion)
“Electron and Positron Fluxes in Primary Cosmic Rays Measured with the Alpha Magnetic Spectrometer on the International Space Station”, M. Aguilar et al., Phys. Rev. Lett. 113, 121102 (2014) (Selected as Editors’ Suggestion).
“Precision Measurement of the (e+ + e-) Flux in Primary Cosmic Rays from 0.5 GeV to 1 TeV with the Alpha Magnetic Spectrometer on the International Space Station”, M. Aguilar et al., Phys. Rev. Lett. 113, 221102 (2014).
“Precision Measurement of the Proton Flux in Primary Cosmic Rays from Rigidity 1 GV to 1.8 TV with the Alpha Magnetic Spectrometer on the International Space Station”, M. Aguilar et al., Phys. Rev. Lett. 114, 171103 (2015) (Selected as Editors’ Suggestion).
“Precision Measurement of the Helium Flux in Primary Cosmic Rays of Rigidities 1.9 GV to 3 TV with the Alpha Magnetic Spectrometer on the International Space Station”, M. Aguilar et al., Phys. Rev. Lett., 115, 211101 (2015) (Selected as Editors’ Suggestion)
“Antiproton Flux, Antiproton-to-Proton Flux Ratio, and Properties of Elementary Particle Fluxes in Primary Cosmic Rays Measured with the Alpha Magnetic Spectrometer on the International Space Station”, M. Aguilar et al., Phys. Rev. Lett., 117, 091013 (2016).
“Precision Measurement of the Boron to Carbon Flux Ratio in Cosmic Rays from 1.9 GV to 2.6 TV with the Alpha Magnetic Spectrometer on the International Space Station”, M. Aguilar et al., Phys. Rev. Lett., 117, 231102 (2016) (Selected as Editors’ Suggestion).
*** EMBARGOED UNTIL 8 DECEMBER 2016, 19:00 Geneva time ***
Unlocking the Secrets of the Cosmos:
The First Five years of AMS on the International Space Station
In a colloquium at CERN today, Professor Samuel Ting of the Alpha Magnetic Spectrometer (AMS) Collaboration presented the results from five years on the International Space Station (ISS).
The AMS Experiment (shown in Figure 1) is the most sensitive particle detector ever deployed in space and is exploring a new and exciting frontier in physics research. As a magnetic spectrometer, AMS is unique in physics research as it studies charged particles and nuclei in the cosmos before they are annihilated in the Earth’s atmosphere. The improvement in accuracy over previous measurements is made possible through its long duration time in space, large acceptance, built in redundant systems and its thorough calibration in the CERN test beam. These features enable AMS to analyze the data to an accuracy of ~1%. The first five years of data from AMS on the International Space Station are beginning to unlock the secrets of the cosmos.
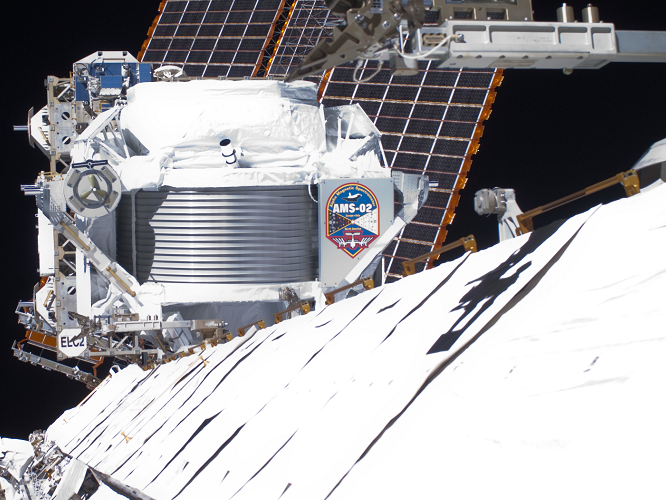
Figure 1. From its vantage point ~240 miles (400 km) above the Earth, the Alpha Magnetic Spectrometer (AMS) collects data from passing cosmic rays from primordial sources in the universe before they pass through the Earth’s atmosphere.
Since its installation on the ISS in May 2011, AMS has collected data from more than 90 billion cosmic rays with up to multi-TeV energies and published its major physics results in Physical Review Letters (Appendix I).
A note about cosmic rays. As the products of exploding supernovae, primary cosmic rays can travel for millions of years in the galaxy before reaching AMS whereas secondary cosmic rays come from the interaction of primary cosmic rays with the interstellar media. Uniquely positioned on the International Space Station, AMS studies cosmic rays passing through its precision detectors, shown in Appendix II, to define the charge, energy, and momentum of the passing particles in order to obtain an understanding of dark matter, the existence of primordial antimatter in space, the properties of primary and secondary cosmic rays as well as new, unexpected phenomena. These are among the fundamental issues in modern physics. Appendix III contains a brief summary of AMS for reference.
There are hundreds of different kinds of charged elementary particles. Only four of them – electrons, protons, positrons and antiprotons – have infinite lifetimes so they can travel through the cosmos indefinitely and eventually reach AMS.
AMS has observed that the electron flux and positron flux display different behaviors both in their magnitude and in their energy dependence. Most surprisingly, from 60 to 500 GeV, positrons, protons and antiprotons display identical momentum dependence but electrons exhibit a totally different dependence as shown in Figure 2. The reason that this observation is surprising is that both electrons and positrons lose energy equally when travelling through the galactic magnetic field and at a much higher rate than protons or antiprotons.
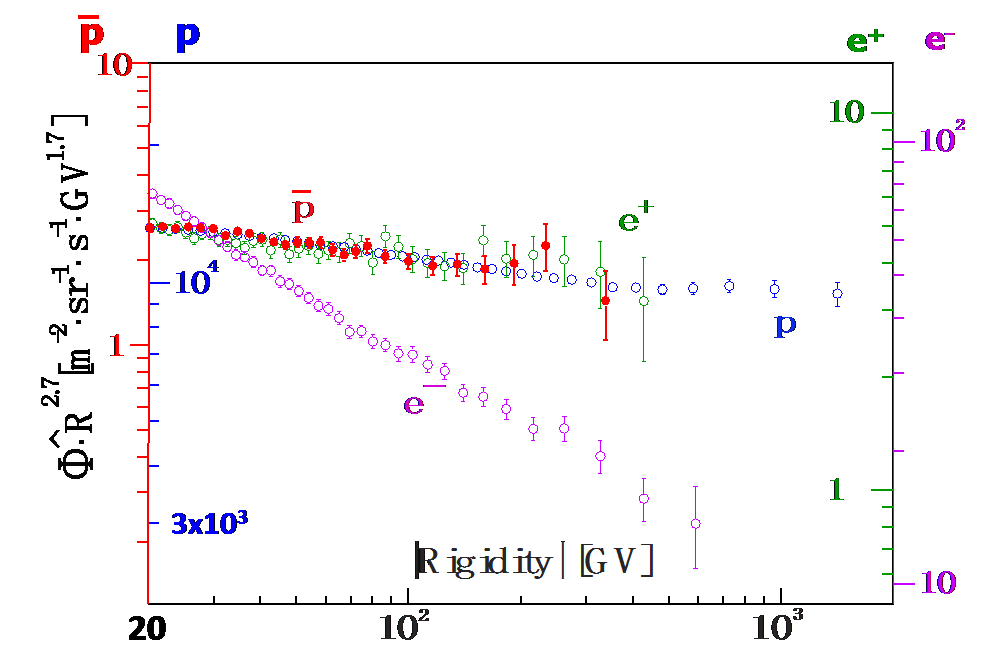
Figure 2. The positron, proton, and antiproton spectra have identical momentum dependence from 60 to 500 GeV. The electron spectrum exhibits a totally different behavior, it decreases much more rapidly with increasing momentum.
There has been much interest over the last few decades in understanding the origin and nature of dark matter. When particles of dark matter collide, they produce energy that transforms into ordinary particles, such as positrons and antiprotons. The characteristic signature of dark matter is an excess of the flux with energy followed by a sharp drop off at the mass of dark matter.
Figure 3 shows the latest results from AMS on the positron flux. As seen from the figure, after rising from 8 GeV above the rate expected from cosmic ray collisions, the spectrum exhibits a sharp drop off at high energies in agreement with the dark matter model predictions with a dark matter mass of ~1 TeV. There is great interest in the physics community on the AMS measurements of elementary particles. For example, an alternative speculation for the measured positron spectrum is that this rise and drop off may come from new astrophysical sources such as pulsars or supernova remnants however they cannot explain other AMS measurements on particles and nuclei.
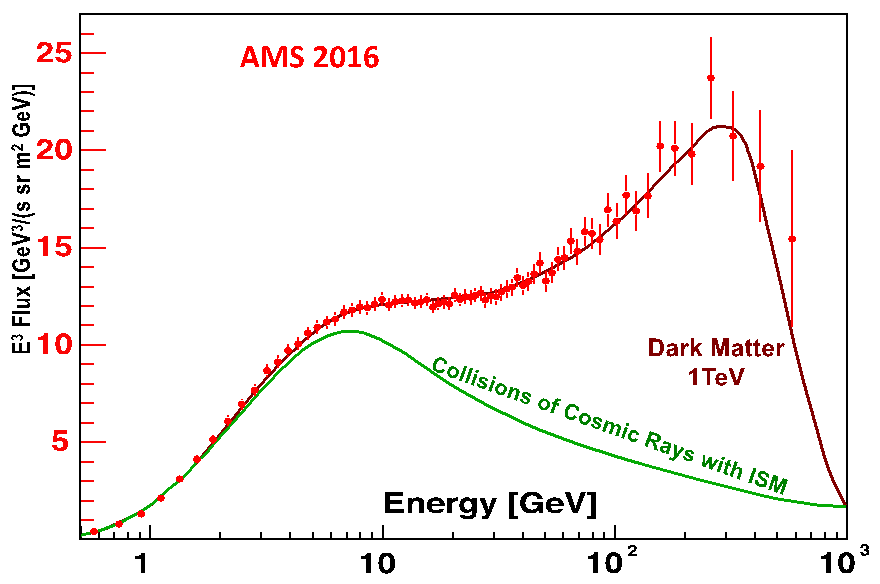
Figure 3. The latest AMS positron flux measurement compared with theoretical models.
AMS has also studied the antiproton to proton flux ratio. The excess in the antiproton flux observed by AMS cannot be explained as coming from pulsars.
Protons are the most abundant particles in cosmic rays. AMS has measured the proton flux to an accuracy of 1% and found that the proton flux cannot be described by existing models.
Helium, lithium, carbon, oxygen and heavier nuclei up to iron have also been studied by AMS. It is believed that helium, carbon and oxygen were produced directly from primary sources in supernova remnants whereas lithium, beryllium and boron are believed to be produced from the collision of primary cosmic rays with the interstellar medium. Primary cosmic rays carry information about their original spectra and propagation, and secondary cosmic rays carry information about the propagation of primary and secondary cosmic rays and the interstellar medium.
Although lithium is a secondary cosmic ray, its spectrum behaves similarly to protons and helium such that none of the three fluxes can be explained by current models.
Since protons, helium, carbon and oxygen are primary cosmic rays and produced at the same sources their flux ratios should be rigidity independent. Rigidity is momentum per unit charge and is the metric by which magnetic fields, such as those experienced by cosmic rays between their origin and AMS, act on charged particles. From the AMS measurements, for carbon-to-helium and for carbon-to-oxygen these ratios are, indeed, independent of rigidity, i.e., flat, as expected. Unexpectedly, the proton-to-helium flux ratio drops quickly but smoothly with rigidity.
Other secondary cosmic rays being measured by AMS include boron and beryllium. The unstable isotope of beryllium, 10Be, has a half-life of 1.5 million years and decays into boron. The beryllium to boron flux ratio increases with energy due to time dilation when the beryllium travels at close to the speed of light. Hence, the ratio of beryllium to boron provides information on the age of the cosmic rays in the galaxy. From this, AMS has determined that the age of cosmic rays in the galaxy is approximately 12 million years.
The flux ratio between secondary cosmic rays (boron) and primary cosmic rays (carbon) provides information on propagation and the average amount of interstellar material through which the cosmic rays travel in the galaxy. Cosmic ray propagation is commonly modeled as a fast moving gas diffusing through a magnetized plasma. Various models of the magnetized plasma predict different behavior of the boron-to-carbon (B/C) flux ratio. Surprisingly, above 65 GeV, the B/C ratio measured by AMS is well described by a single power law B/C=kRd with d = -0.333±0.015. This is in agreement with the Kolmogorov turbulence model of magnetized plasma where d = -1/3 asymptotically. Of equal importance, the B/C ratio does not show any significant structures in contrast to many cosmic ray models used to explain the excess in the positron flux measured by AMS.
The AMS data also shows that the carbon and oxygen fluxes, which are both primary cosmic rays, and the boron, lithium, and beryllium fluxes, which are secondary, have characteristically different momentum (or rigidity) dependences.
The Big Bang origin of the Universe requires that matter and antimatter be equally abundant at the very hot beginning of the universe. The explanation for the apparent absence of primordial antimatter is known as Baryogenesis. Baryogenesis requires both a strong symmetry breaking and a finite proton lifetime. Despite the outstanding experimental efforts over many years, no evidence of strong symmetry breaking nor of proton decay have been found. Therefore, the observation of a single anti-helium event in cosmic rays is of great importance.
AMS has collected 3.7 billion helium events (with positive charge). To date we have observed a few negatively charged events with masses around 3He. At a rate of approximately one antihelium candidate per year and a required signal (antihelium candidates) to background (helium) rejection of one in a billion, a detailed understanding of the instrument is required. In the coming years, with more data, one of our main efforts is to ascertain the origin of the antihelium candidates.
In five years on the Space Station, AMS has provided accurate information on elementary particles and nuclei and is beginning to unlock the secrets of the cosmos.